Featured in
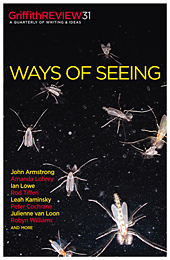
- Published 20110301
- ISBN: 9781921656996
- Extent: 264 pp
- Paperback (234 x 153mm), eBook
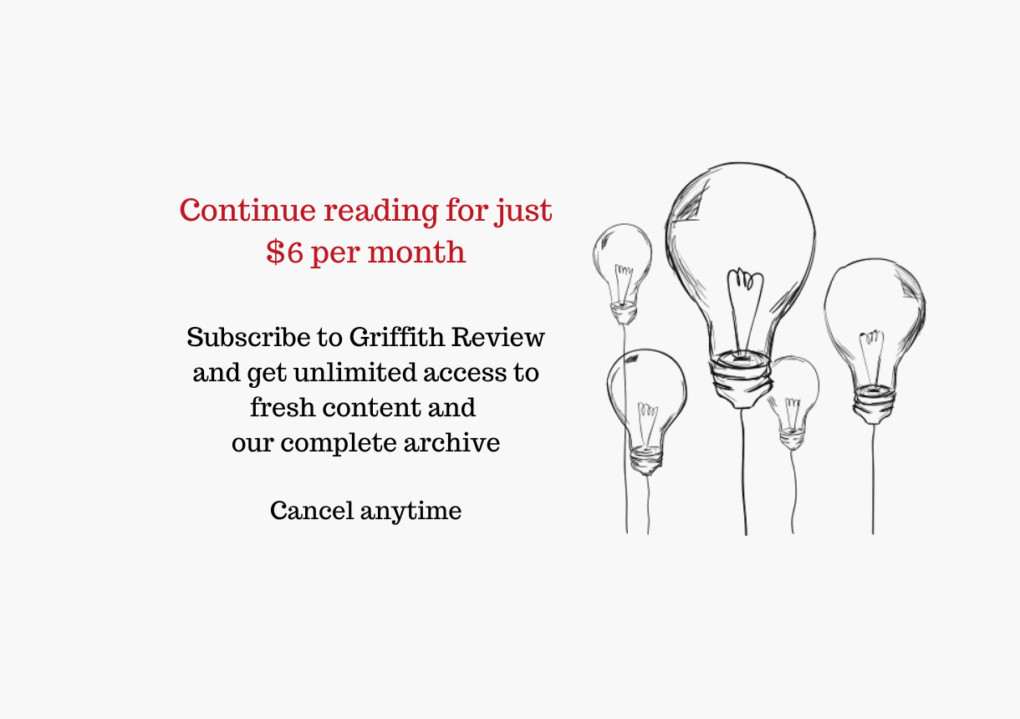
Already a subscriber? Sign in here
If you are an educator or student wishing to access content for study purposes please contact us at griffithreview@griffith.edu.au
Share article
More from author
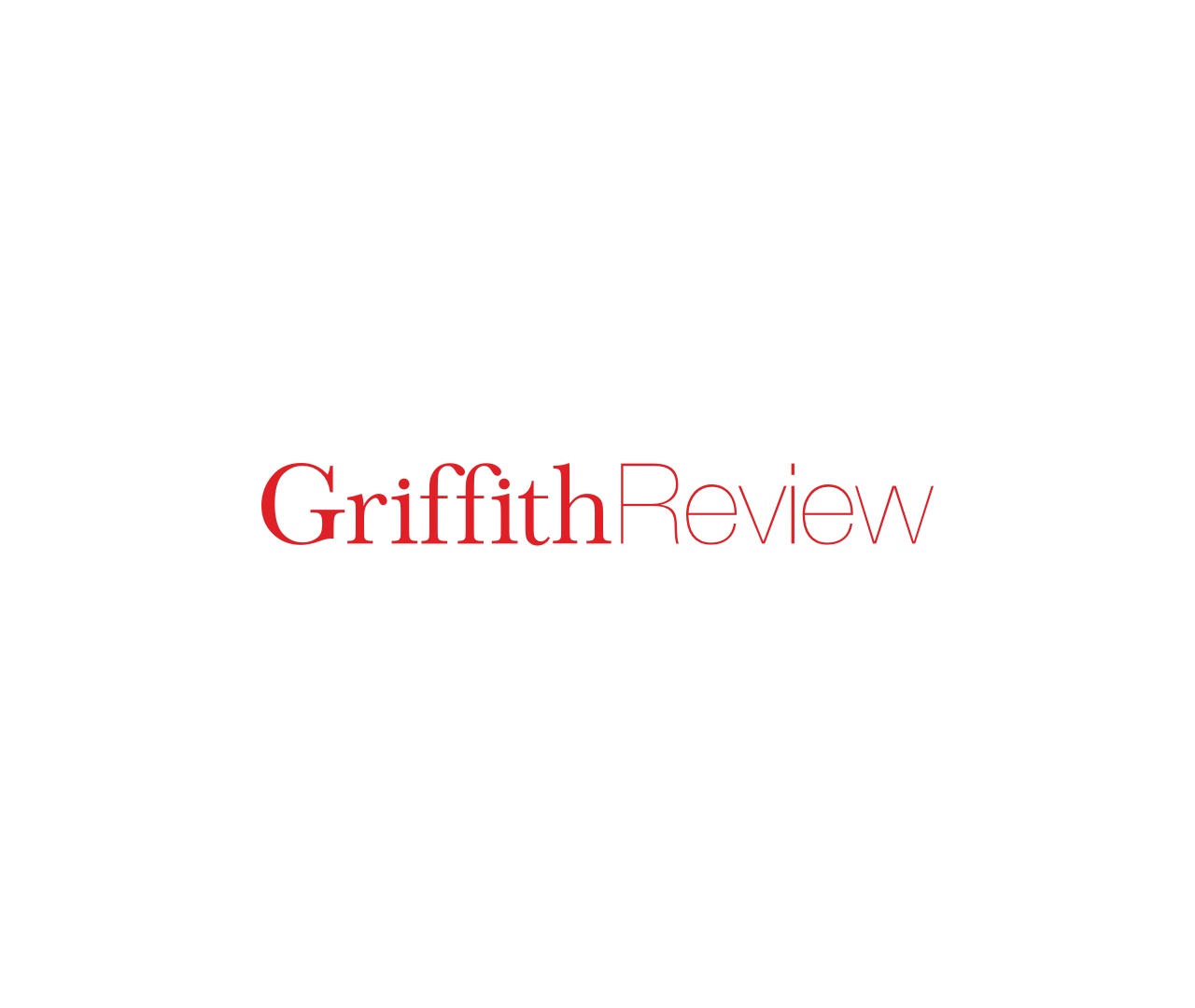
A long half-life
EssayON MY DESK there sits a well-thumbed copy of the 1976 Fox Report, the first report of the Ranger Uranium Environmental Inquiry. I grew up...
More from this edition
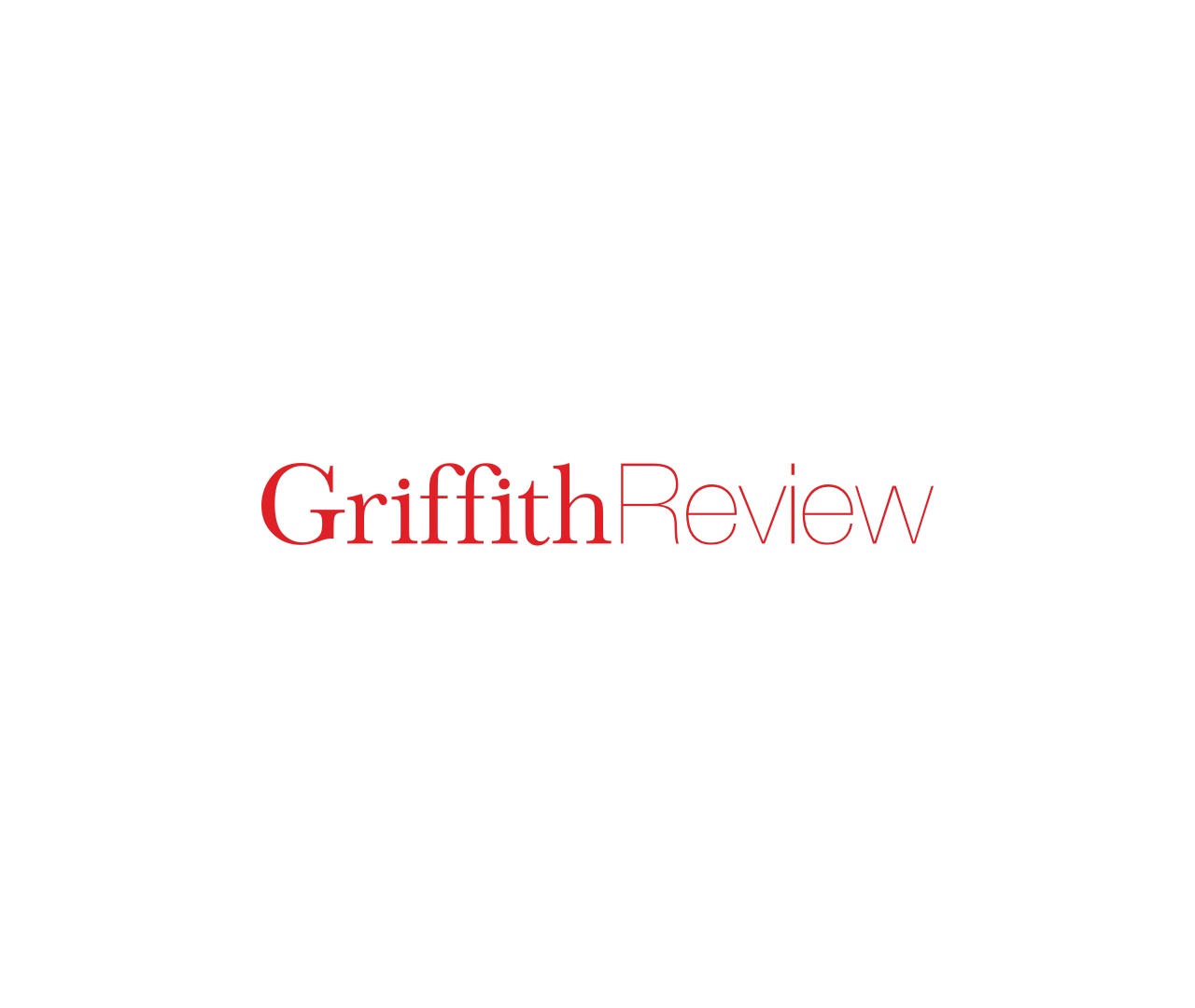
In praise of experts
IntroductionWALKING ACROSS THE scorched lawns of the quadrangle of a major university, not so long ago when this was a land beset by drought...
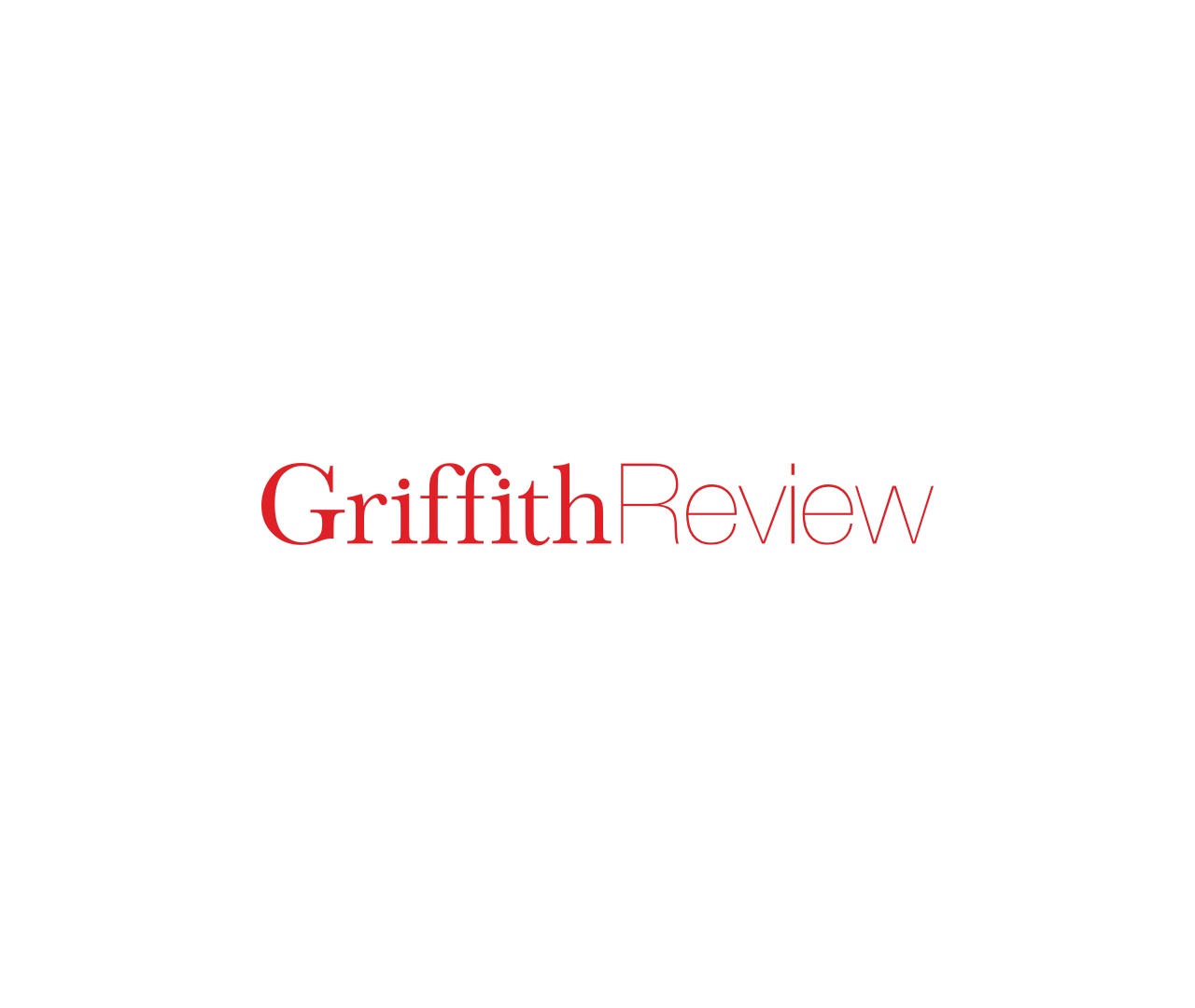
Your job is to amaze me
GR OnlineI'M ABSOLUTELY DELIGHTED to have been invited out of retirement to have the privilege of addressing you. I only draw attention to my 'retirement'...
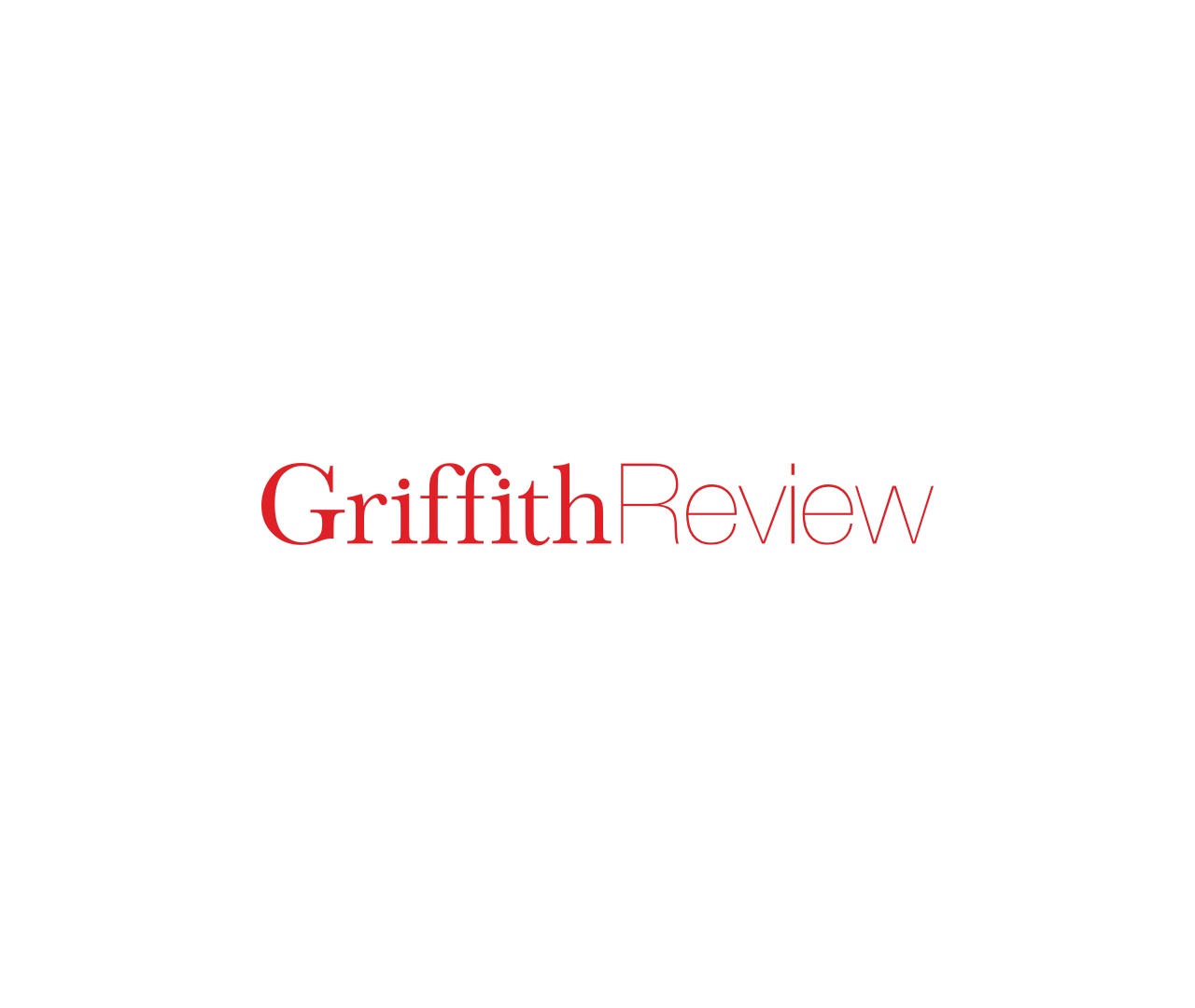
The lords of time
GR OnlineTHOMAS MORE'S UTOPIA 1516 was recently claimed as the most influential fiction in history, that it had worked its way out of simply being...