Featured in
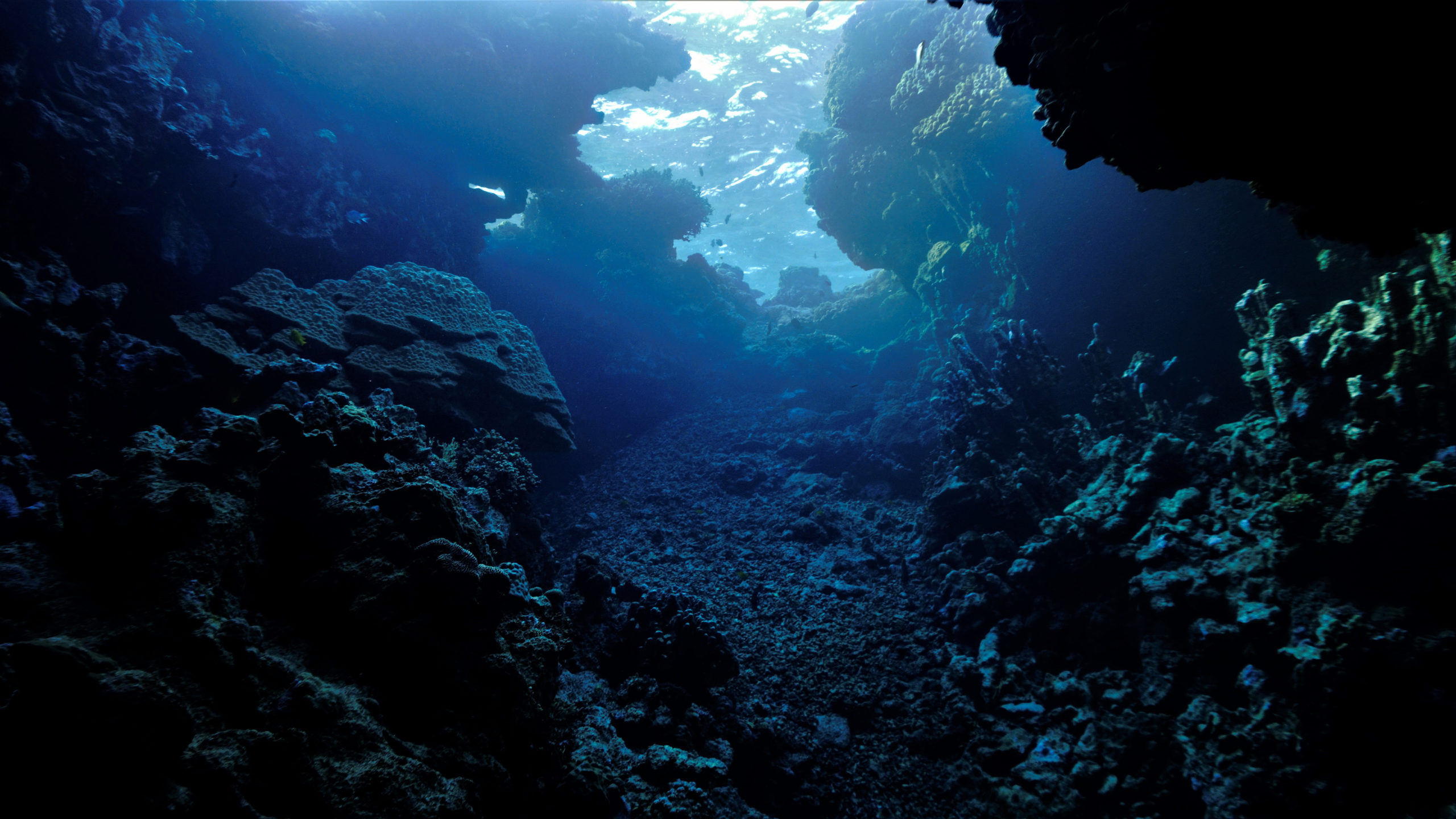
IN MY TWENTIES and thirties I spent as much time as I could in the waves off Sydney’s beaches. Having come to surfing late, I was next to useless on a board, and while fun, boogie boarding always seemed a little undignified. So instead my brother, a few friends and I took to using hand–skis to catch the swells that crash in from the Pacific.
Hand–skis (or pods or handboards – the terminology seems to have more to do with who makes them than the basic technology) are exactly what they sound like – curved planes or blades about the size of a dinner plate that are strapped to your hand and act as a hydrofoil. Using one is intensely exhilarating, its small surface lifting your head and shoulders out of the water as you shoot down the face of the wave, your body suddenly seeming as sleek and manoeuvrable as a dolphin’s or a seal’s.
The catch is that such rides aren’t possible in the mess of the shore break. And so we took to swimming out into the deeper water, beyond the back break. Out there, we would take on waves twice our height – great green and blue slabs of curving water that, if caught right, would send us hurtling beachward until we slipped out the side or were swallowed by the spume. It was exhilarating, not least because it offered a reminder of the sheer power of the ocean: cut it too close and the weight of the wave’s collapse behind you would detonate like something seismic – an atom bomb, we used to call it, only half-jokingly – but if we mistimed an exit or got caught in the dump zone, excitement could quickly give way to fear.
Yet in many ways what really interested me was not the adrenaline rush of riding the waves, but the quieter moments. Because out there, as the swell moved beneath me, slow and steady as breath, it was sometimes possible to feel the intimation of something larger, a sense of time’s depth, of the great pulse of the world’s cycles. And although it was never easy to articulate, it sometimes seemed that something transformative inhered in those moments, as if to give oneself over to the ocean’s largeness and the movement of the wind and the waves might offer a glimpse of another way of being.
This sense of boundlessness is a frequent theme among those who spend time in the water. Australian author and surfer Fiona Capp writes of the ‘inkling of infinity’ in the face of the ocean’s immensity and power, observing that ‘few images capture this primal “at oneness” than that of the surfer crouched inside the crystal, womb-like tube of a breaking wave; an image made all the more exquisite by our knowledge of the wave’s imminent destruction’. Capp notes this moment of suspension and inevitable ejection from the ‘all-embracing amniotic realm’ of the wave’s oneness is also embedded in the Hawaiian word for surfing, he’e nalu, or ‘wave-sliding’. ‘He’e means “to run as a liquid” or “flee through fear”, while nalu refers to the surging motion of a wave or the slimy liquid on a newborn child.’ Free diver James Nestor says something similar, suggesting that ‘letting the ocean envelop you’ can be ‘a spiritual practice, a way of using the human body as a vessel to explore the wonders in the Earth’s inner space’.
Sigmund Freud’s friend, the writer Romain Rolland, called this apprehension of the infinite and sense of oneness with the universe oceanic feeling, and argued it was the source of religious feeling. Freud – who said he found no scrap of this sentiment in himself – was more circumspect, contending (in a suggestive echo of the Hawaiian association with gestation and birth) that if it did exist, it was a vestigial remnant of early infancy, and our still egoless and undifferentiated self’s inability to distinguish between the world and ourselves.
More recently, scientists and psychologists have found evidence these feelings of expansive awareness have a neurological origin, connecting them to the interaction between the neural network our brains use when focusing on the external world and that which governs internal processes relating to self-reflection, self-awareness and emotion. Normally these two networks operate independently, but in a 2011 study of the brains of Buddhist monks, neuroscientist Zoran Josipovic discovered that when meditating it was possible to use both networks simultaneously, thereby lowering the barrier between the self and the environment, and inducing a sense of expansive awareness and connection to the world. As writer Wallace J Nichols has observed, these same feelings are often found in activities such as surfing and skydiving that demand an intense focus on the immediate; in these moments it often seems impossible to distinguish between the world and ourselves: ‘when we access these states we see ourselves not as separate but as “embedded” in our relationships with everything in the world; we are part of everything, and everything is a part of us.’
It is perhaps little wonder, therefore, that we so often invoke the water or the ocean when we speak of origins, or time. As the historian Fernand Braudel recognised when he chose the marine metaphor ‘deep time’ to describe those cycles of history that exceed the human – and stretch downwards, into the geological – the depth and mystery of the ocean speak to our sense of the boundless and the infinite. Nor is it an accident that, as philosopher Ivan Illich reminds us, so many of our Creation stories begin with an act of division, with the conjuring of form from the primal unity of water. Or that we so often blur the boundaries between the self and the aqueous, whether in Burton’s description of the ‘tides of melancholy’, other everyday expressions such as ‘waves of feeling’, ‘drifting off’ and ‘bodies of water’, or more contemporary notions such as creative ‘flow’. Likewise water’s religious significance is immense: for Hindus the Ganges is sacred, its waters cleansing those who bathe in them of confusion and sin, much as the Christian ritual of baptism washes away sin. Simultaneously water is also associated with dissolution, unmaking and rebirth. For the Greeks the River Lethe was the river of forgetfulness, while the Styx formed the boundary between the world of the living and the Underworld, just as in Egyptian mythology the souls of the Pharaohs travelled up the Nile after death.
It is possible this association with water goes back even further. Although few scientists take seriously the aquatic ape theory that postulates our ancestors returned to the water for a time, there is no question our bodies remember other, older ways of being that connect us to the water. We bear the ocean in our veins, in the salt of our blood, in our genetic memory of life’s birthplace. Placed in water a human infant will instinctively hold its breath and begin to breaststroke, a response that only fades when we learn to walk. Adult humans have similar abilities hidden deep in our physiology. Slip beneath the water’s surface and your body immediately begins to change, your heart slowing as your blood moves from your extremities to your core, reducing your consumption of oxygen to prolong your life and protect your organs. Sometimes referred to as the master switch of life, or the mammalian diving reflex, these adaptations are shared with seals and other marine animals; those who know how to use them are capable of swimming to great depths without suffering the ill effects of nitrogen narcosis or the bends.
In recent years these intuitions have been given new dimension by the work of cultural theorists such as Astrida Neimanis, who have explored the idea of a ‘hydrological turn’. For Neimanis, the liquidity of our bodies becomes a way to reimagine our most fundamental ideas about embodiment and individuality, reminding us that our bodies and selves are always in flux, as water moves in and out of us, and connecting us not just to each other, but to other organisms and the larger cycles of the planet. Meanwhile, in what are known as the Blue Humanities, scholars argue for the importance of oceanic thinking, of recognising the ocean’s role not just as a place of making and unmaking, of transformation and change, but as a medium of interconnection and entanglement, the consideration of which affords us important new perspectives on human history, planetary time and – perhaps most profoundly – our relationship to the world as a whole.
WATER IS EVERYWHERE on Earth; life is inconceivable without it. Yet the origins of this water are complex and mysterious. To understand them we must go back almost fourteen billion years to the Big Bang itself. In the moments after the first expansion that created the universe, the first protons and neutrons began to form and, as the universe continued to expand and cool, these gradually began to collide to form atoms. Most of these took the form of hydrogen atoms – then, as now, the simplest and most abundant element in the universe; less frequently they were atoms of helium or lithium.
For a long time this matter was spread through the still rapidly expanding universe in a thin cloud. But over time, clumps began to form. As they collapsed in on themselves they grew larger and denser, until finally the pressure at their centre caused them to ignite, and in the nuclear furnace at the hearts of these first stars atoms began to fuse together, releasing vast amounts of energy.
At first this process mostly created more helium. But as the stars aged, more complex elements began to be produced, as atoms were fused together into more and more complex arrangements. Sometimes these new elements were as heavy as iron, but most of what was produced was oxygen, so much in fact that it gradually became the third most abundant element in the universe. As it was released, this oxygen fused with the even more abundant hydrogen, and formed water. This process continues even today: in the Orion Nebula enough water is created every day to fill Earth’s oceans sixty times over.
The next stage begins with the formation of our own solar system, just over 4.5 billion years ago. The solar system began its existence as one part of a vast nebula, which at some point began to collapse inwards and rotate, drawing material inward, until it formed the protostar that would become our sun. Meanwhile the remaining matter, now spread out in what is known as the protoplanetary disc, began to cohere also, clumping together into larger and larger units, eventually forming small planetesimals, and eventually planets.
The distance of these new planets from the sun determined their composition. Close to the sun, where the heat was so great neither water nor methane could condense, they formed out of metals and silicates, creating the small, rocky planets that now populate the inner solar system; further out, beyond what astronomers call the frost line, they formed out of ice and gases borne outward on the solar wind.
This earliest period of the Earth’s existence – known as the Hadean – was unimaginably violent. Temperatures exceeded 1,000 degrees Celsius, radioactivity was high, and the atmosphere was a toxic mixture of ammonia and methane. The Earth’s surface was molten, a highly unstable lake of magma, and even after a crust began to form, was subject to volcanic eruptions and frequent impacts by meteors and other debris. The most violent of these – the collision with the Mars-sized planet Theia fewer than one hundred million years after the creation of the solar system – was so catastrophic it created the Moon.
For many years it was assumed the Hadean Earth was so hot that any water captured during its formation would have simply boiled away. As a result, astronomers proposed the vast bulk of our planet’s water arrived later, borne to Earth by a barrage of water-bearing meteorites and comets between 3.8 and four billion years ago.
Yet in recent years this theory has come under increasing scrutiny. As many astronomers have noted, the moon’s surface shows no evidence of such a bombardment. Likewise, some models suggest that the number of impacts that would have been needed to deliver the water and other elements would have caused the Earth’s crust to remelt. And, perhaps even more problematically, while the atomic signature of the water contained in certain meteorites matches that of the water on Earth, the water in many comets does not, meaning they cannot have been the main source of our planet’s water.
A solution to this conundrum might lie in geological samples extracted from the Jack Hills in Western Australia. Located some 300 kilometres inland from Shark Bay, the rocks that comprise the Jack Hills are extraordinarily ancient, having formed some 3.6 billion years ago. Yet analysis of samples taken from the western part of the range in the 1980s have been found to contain minute zircon crystals that are even older, dating back an astonishing 4.4 billion years.
The Jack Hill zircons are the oldest known remnants of our planet’s primordial crust – or in layperson’s terms, the oldest rocks on the planet – so old, in fact, that they formed a mere 160 million years or so after the Earth itself. This would be remarkable enough on its own, yet more tantalisingly, analysis of their chemical composition shows they must have formed somewhere wet, suggesting not just that there must have been water present on Earth not long after its creation, but that the Earth’s crust must have cooled sufficiently for this water to condense much earlier than previously thought.
The presence of liquid water doesn’t mean this early Earth was particularly cool, or hospitable to life: in fact surface temperatures would have been close to 200 degrees, and the water was only prevented from boiling away by the extremely high atmospheric pressure. But what it does suggest is that at least some of Earth’s water was acquired when the planet first began to form out of the protoplanetary disc, and somehow survived not just the heat of the Hadean Earth, but the collision that formed the Moon.
Further support for the idea water may have been present in this primordial Earth emerged in 2014, when scientists detected a vast reservoir 700 kilometres beneath the Earth’s surface, perhaps containing several times as much water as that which exists on the surface. It is further supported by the discovery of what appear to be fossils of microbes dating back almost 4.3 billion years. Closely resembling organisms that live on hydrothermal vents in the deep ocean today, these fossils suggest life on our planet began much earlier than was previously believed possible.
That these theories continue to evolve and change underlines just how dynamic our understanding of the Earth and our solar system’s history remains. Yet what seems clear is that Earth’s water – and by extensions its oceans – have multiple sources. Some clearly survived the Hadean, and even the collision with Theia. But some also came from space, borne in from the outer reaches of the solar system by comets. Indeed this process continues even today – as comets swing in towards the sun they release dust and water vapour, creating their distinctive tails; once released this water freezes again, leaving a fine sprinkling of ice drifting in the comets’ wake. And as it makes its way around the sun, the Earth sweeps up this snow; each year 30,000 tonnes of water still drift earthward in this way, a gentle rain of cometary material that is absorbed into our atmosphere, our rivers and oceans. Our bodies.
EARTH IS NOT the only world with water. On Jupiter’s moon, Europa, a vast ocean perhaps one hundred kilometres deep containing more than twice as much water as Earth’s oceans is believed to lie between the moon’s rocky centre and its frozen surface. Kept liquid by the heat created by the tidal force Jupiter’s immensity exerts upon Europa, this ocean’s dark waters are believed to be warm and salty, like our own oceans, like our blood. On Saturn’s moon, Enceladus, a similar ocean lies beneath an ice sheet some thirty or forty kilometres thick; its waters escape through ice volcanoes at the moon’s south pole in huge geysers that rise hundreds of kilometres into space. Most of the water released in these plumes drifts back onto the surface of Enceladus and freezes again. That which does not is lost to space, mostly coming to rest in Saturn’s rings.
Although the oceans on Europa and Enceladus are the most famous, they are not the only instances of such ice worlds among the many moons and dwarf planets scattered through the outer reaches of the solar system. Jupiter’s largest moon, Ganymede, may hide an ocean even bigger than Europa’s, possibly bound up in concentric layers of ice and liquid water, and oceans are also believed to exist beneath the icy surfaces of Callisto, Dione and tiny Mimas as well as even more distant moons such as Uranus’ Ariel and Neptune’s Triton. Even Titan, the surface of which is so cold there are sandhills of frozen hydrocarbons and seas of liquid methane, is believed to hide an underground ocean with water as salty as that of the Dead Sea on Earth. Even Pluto – so far from the sun that its light takes five hours to reach it – may possess an ocean beneath its icy surface.
These frozen worlds out beyond the frost line bear little resemblance to Earth and our neighbours in the inner solar system. Yet even in here, close to the sun, there is evidence of ancient oceans. Although Mars is now barren and hostile, four billion years ago it possessed abundant surface water, probably concentrated in two major bodies: a vast, shallow ocean that covered the northern third of the planet, and another, smaller sea in the south.
What happened to Mars’ oceans is not fully understood. What we do know is that Mars lacks the magnetic fields that protect Earth, meaning its atmosphere must have been gradually stripped away by the solar wind. As atmospheric pressure fell, Mars’ surface water froze or was sublimed away; that which was not lost sank into the surface, freezing solid, combining with chlorate and perchlorate salts to form the brines that even today seem to flow occasionally on the Martian surface, or gathering in underground reservoirs like those recently discovered beneath Mars’ southern pole.
Venus was also once a very different place. Although closer to the sun than Earth, Venus’ size and composition are so similar to Earth’s that they might almost be sisters. Yet unlike Mars, whose barren surface was able to be studied long before the advent of spaceflight, the clouds that envelop Venus meant its surface remained tantalisingly unknowable until the first spacecraft visited it in the 1960s.
Faced with this mystery, astronomers imagined a world that was hot and wet and swathed in heavy cloud. In the early twentieth century the Swedish scientist Svante Arrhenius (who was also the first to discover the cycle of Earth’s climate was connected to the changing concentrations of carbon dioxide in the atmosphere) suggested Venus’ clouds were water vapour, and that beneath them the planet was both extremely warm and extremely humid. Predicting its surface would be covered by swamps and oceans, he imagined conditions rather like those on Earth during the Carboniferous era, when much of the vast deposits of coal and oil on which we now rely were laid down, and speculated its seas and jungles might be home to abundant but simple plant life, fast-growing and quickly decaying.
Yet when probes finally visited the planet in the 1960s, they did not find lush rainforests and warm seas but a horrifying hellscape where surface temperatures average more than 450 degrees Celsius, and an incredibly dense atmosphere composed almost entirely of carbon dioxide trapped beneath heavy clouds of sulphuric acid. Atmospheric pressure is ninety-two times that of Earth’s at sea level, or equivalent to almost a kilometre beneath the ocean, so dense in fact that early probes simply stopped functioning well before they reached the surface.
Yet if the unimaginable hostility of Venus was a shock to scientists, it was perhaps less of a shock than the discovery it had not always been how it is today. Instead, early in its history, Venus boasted conditions similar to those on Earth, up to and including the presence of liquid water in the form of oceans. At some point it grew warmer, and the water began to evaporate, combining with the carbon dioxide and methane already present in the atmosphere to act as a blanket, trapping more and more of the sun’s heat and triggering a feedback loop that caused a runaway greenhouse effect that eventually boiled away 99.95 per cent of Venus’ water to create the hellish conditions we see today.
Exactly why this happened is not clear. Until recently it was assumed it was connected with a gradual increase in the sun’s intensity, but in 2020 scientists from NASA’s Goddard Institute presented findings suggesting Venus may have continued in a relatively Earth–like state until as recently as about 750 million years ago, at which point a sudden spike in carbon dioxide – perhaps as the result of a volcanic event such as the explosion of the Siberian Traps here on Earth – triggered the runaway greenhouse effect. If their assumptions are correct, that means Venus may have had oceans – and possibly even life – until fewer than a billion years ago.
It is, of course, possible to see in the stories of Mars and Venus other versions of our own planet’s history, alternative scenarios in planetary evolution. Yet they also remind us not just of the importance of water and the oceans to life on our planet, but of the fragility of those processes, their improbability and impermanence.
JUST OVER FIFTY years ago, when the astronauts of the Apollo program left low Earth orbit for the first time, they looked back, and took a photo of something no human had ever seen before. This photo – dubbed ‘Earthrise’ – captured our planet suspended above the lifeless surface of the moon, vivid and alive in the inky void of space. Together with the famous Blue Marble photo taken a few years later by Apollo 17, the Earthrise photo changed the way we saw our world, revealing our planet not as a collection of countries or continents, but as one vast, interconnected system, which we all share. As astronaut William Anders famously remarked, ‘We set out to explore the Moon, and instead discovered the Earth.’
Yet this understanding was complemented by another, which is that seen from space, our world is a blue planet. Perhaps this should not come as a surprise: after all, the oceans cover more than 70 per cent of the Earth’s surface, and the biggest of them – the Pacific – is larger than all the continents combined. Yet the Apollo images made these sorts of statistics graspable in a way they had not been before, capturing not just how the oceans dwarf the planet’s land masses, but the shattering whiteness of the polar ice, and the beauty and complexity of the ribs and swirls of the massing clouds.
Indeed water has shaped our planet since its molten surface first began to cool, 4.5 billion years ago. Rain, rivers and waves weather its crust and transport heat and minerals from the Earth’s mantle to the surface, shaping its geology and creating soils. Water also drives the complex web of processes known collectively as the hydrological cycle, as evaporation from the oceans and elsewhere returns to the surface in the form of rain and snow, where its movement transports sediments and sustains plants and animals, before evaporating once more. Similarly the vast engine of thermohaline circulation in the oceans transports heat from the tropics to the poles, shifting warm water pole–wards and bringing cooler water toward the equator, regulating the climate and helping drive the systems of wind and current that shape the planet’s climate. So immense is this system that it can take up to 1,000 years for some of this water to make the journey there and back.
These systems have also shaped human society, determining the climate and creating the ocean corridors that laid the foundations for the world we now inhabit. The Atlantic slave trade was made possible by the two vast systems of wind and ocean currents that dominate the North and South Atlantic, turning in opposite directions on each side of the Equator like two vast wheels. Using the clockwise motion of the system in the North Atlantic, goods flowed from England and Northern Europe to Northern Africa, where they were exchanged for human beings, who were then carried west, across the Atlantic to the Caribbean and the Americas, before the ships returned to England and other northern European ports laden with the sugar, tobacco and cotton produced on the slave plantations. Meanwhile, in the Southern Hemisphere, anticlockwise currents carried Portuguese slavers back and forth between West Africa and Brazil. Patterns of wind and current also made possible the British invasion of Australia and New Zealand, as the ships followed the trade winds to the Southern Ocean, where they took advantage of the winds that sweep around the globe in the roaring forties to travel eastward. And in the Pacific, shifting wind patterns enabled the settlement of far-flung islands such as Rapa Nui, before isolating them again when weather patterns changed once more.
Yet while the ocean’s immensity often leads us to think of it as limitless, it is not. Over recent decades humans have released hundreds of billions of tonnes of greenhouse gases into the atmosphere. The vast bulk of the heat trapped by these gases has been absorbed by the oceans, and most of that by the top few hundred metres. This has had a dramatic effect on the rate of global heating: indeed without the insulating effect of the oceans, global temperatures would have risen by well over half a degree more over the past fifty years. But this heat has not just disappeared: records show temperatures in the top twenty metres of the ocean have increased by an average of 0.13 degrees a decade over the past century. Even more alarmingly, this process is accelerating: one recent study showed not just that the oceans warmed 4.5 times faster between 1987 and 2019 than they did between 1955–86, but that ocean temperatures in 2019 – already at a record high – had increased by the largest amount in a decade.
These rising temperatures have had dramatic consequences on life in the ocean, disrupting food chains, damaging ecosystems, lowering oxygen levels and leading to increasingly extreme weather, marine heatwaves and coral bleaching events such as those that have devastated reefs around the world in recent years. And this process of change will only speed up as ocean temperatures continue to rise, leading to yet more extreme weather, increasing stress and disruption to marine life and, eventually, to large-scale ecosystem collapses as coral reefs disappear and coastal environments are affected by rising sea levels. Even the water itself is changing and growing more acidic, as the increased amount of carbon dioxide it is absorbing alters its chemical composition.
Other water sources are equally stressed. In higher altitudes and at the poles the cryosphere is changing rapidly as glaciers melt and sea ice disappears. As I write this in November 2020, sea ice in the Arctic is at a record low, and in the Laptev Sea the water has not yet begun to freeze, long after this process should have begun. Freshwater ecosystems are also in crisis. Assailed by irrigation and pollution and drought, populations of freshwater animals have fallen by a staggering 84 per cent over the past fifty years, and left one–in–three freshwater species threatened with extinction. Water scarcity is also affecting human communities in sub-Saharan Africa and elsewhere, and this pressure will only intensify as temperatures continue to rise over coming decades. As the example of Syria makes clear, the human cost of this process will be immense, driving increased levels of conflict and violence and displacing growing numbers of people. Or, as Naomi Klein has observed, ‘Just as bombs follow oil, and drones follow drought, so boats follow both: boats filled with refugees fleeing homes…ravaged by war and drought.’
IN FIVE BILLION years’ time, the sun will burn through the hydrogen at its core and begin to expand, swelling outwards and transforming into a red giant. As it grows, it will swallow Mercury and Venus, swelling outward to beyond the current orbit of the Earth. Our planet may escape being consumed, released into a larger orbit by the weakening of the Sun’s gravity, but even if it does, life will already be gone, perhaps as soon as a billion years from now, when the sun’s heat will have increased enough to trigger a runaway greenhouse effect similar to that which transformed Venus 700 million years ago. Subjected to the sun’s heat and radiation, Earth’s water will be stripped away, broken down into its constituent atoms and borne away on the solar wind.
We are connected to that deep future, just as we are connected to the deep past, bound to it by the water of which we are made, the water that surrounds and flows through us, its continuity extending outward through time as well as space, a reminder of time’s immensity and of the extraordinary, impossible complexity of the web of processes that sustain our lives. Yet the shared liquidity of our bodies is also a reminder that we cannot separate ourselves from the world around us, or the wave of extinction and disaster human activity has sent washing across the planet. Recognising this continuity can be unsettling, even terrifying, demanding we grapple with the impact of our lives and choices on the existence of billions of other human and non-human existences to which they are connected, and to the historical processes that have shaped those webs of connection. Yet like the feelings of oneness we sometimes feel in the ocean and elsewhere suggest, perhaps it might also be transformative, a gateway to a new and better way of being.
Share article
More from author
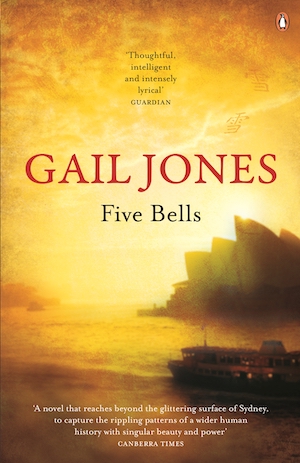
On ‘Five Bells’, by Gail Jones
EssayGAIL JONES’ FIFTH novel, Five Bells, is many things: a love letter to Sydney and its physical beauty; a deeply moving exploration of the effects of grief and loss; and, perhaps most importantly, a luminous and shimmering reflection on time, memory and mortality.